
In today’s rapidly warming world, the quest to curb escalating carbon emissions has never been more critical. Amidst a plethora of strategies to combat climate change, Carbon Capture and Storage (CCS) stands out as a beacon of hope and innovation. This technology, which allows for the capture of carbon dioxide emissions from sources like power plants and industrial processes and the subsequent storage of this CO2 underground, is pivotal in our global efforts to mitigate the environmental impacts of human activity.
The importance of CCS cannot be overstated, especially in light of the ambitious targets set by international agreements such as the Paris Agreement, which aims to limit global warming to well below 2 degrees Celsius above pre-industrial levels. Achieving such a feat necessitates a multifaceted approach, of which CCS is a crucial component. It represents one of the few current technologies capable of significantly reducing emissions from heavy industries and power generation, sectors where alternative reduction strategies are limited or costly.
However, the deployment of CCS technologies is not without its challenges. High implementation costs, technological readiness, and public acceptance are but a few hurdles that need to be overcome. Recognizing these obstacles, various international bodies and agreements have started to lay the groundwork for wider CCS adoption. For instance, the Clean Energy Ministerial’s CCUS Initiative and the Carbon Sequestration Leadership Forum (CSLF) are instrumental in fostering global cooperation and knowledge sharing in CCS development and deployment.
Moreover, carbon pricing mechanisms, including carbon taxes and cap-and-trade systems, have emerged as effective tools in making CCS investments more attractive. By putting a price on carbon emissions, these policies encourage companies to reduce their carbon footprint, with CCS being a viable strategy for compliance. Alongside, tax incentives such as the United States 45Q tax credit for carbon sequestration highlight the role of fiscal policies in lowering the financial barriers to CCS deployment.
As the world strives to meet the Paris Agreement goals, the role of CCS in mitigating climate change gains prominence. According to the International Energy Agency (IEA), to achieve net-zero emissions by 2050, CCS capacity must see a significant increase, highlighting the urgent need for accelerated development and deployment of these technologies.
A tapestry of policies and case studies from leading nations marks the global CCS landscape. The United States, with its robust 45Q tax credit and substantial Department of Energy funding, stands as a pioneer in CCS research and project development. Norway’s investment in projects like Sleipner and Northern Lights showcases its commitment to leveraging CCS for carbon neutrality. Similarly, Canada and the United Kingdom are making strides with regulations and funds aimed at bolstering CCS deployment within their borders.
Carbon Capture and Storage (CCS) technologies represent a critical juncture in our collective climate action efforts. With the backing of international agreements, supportive policies, and global cooperation, CCS technologies offer a pathway to significantly reduce greenhouse gas emissions, paving the way for a sustainable future. Yet, the journey is complex, requiring concerted efforts from governments, industries, and communities worldwide to overcome the challenges and harness the full potential of CCS in our fight against climate change.
THE INTERNATIONAL AGREEMENTS
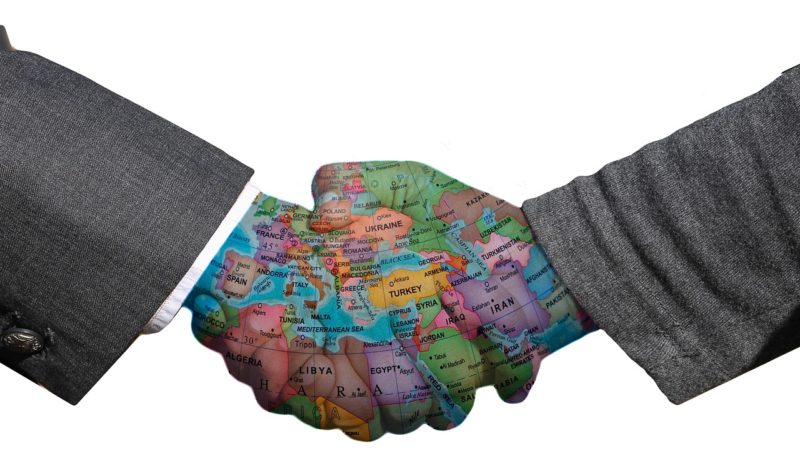
International agreements are the bedrock upon which the global community constructs its collective response to climate change. These compacts, forged through negotiation and consensus among nations, set the stage for coordinated action and shared goals in the fight against global warming. At the heart of these efforts lies an increasing recognition of Carbon Capture and Storage (CCS) technology as an essential tool in the arsenal against rising temperatures. As the world seeks to navigate the complex challenge of reducing greenhouse gas emissions while fostering economic growth and energy security, international agreements provide a framework for integrating innovative solutions like CCS into global climate strategy. By establishing targets, promoting cooperation, and facilitating the exchange of knowledge and resources, these agreements aim to harness the collective capacity of the international community to mitigate the impacts of climate change and steer the planet towards a more sustainable and resilient future.
Paris Agreement
The Paris Agreement, adopted in 2015, marks a global consensus to combat climate change by limiting global warming to well below two °C, aiming for 1.5°C above pre-industrial levels. CCS plays a critical role in this ambition, as it offers a pathway to reduce greenhouse gas emissions from industrial and energy sectors significantly.
The agreement encourages countries to incorporate CCS in their Nationally Determined Contributions (NDCs), essentially national plans to reduce emissions and adapt to climate impacts. The inclusion of CCS in NDCs underscores the significance of technology in achieving long-term climate goals.
Clean Energy Ministerial (CEM) and the CCUS Initiative
The CEM’s CCUS Initiative is a prime example of international cooperation to advance CCS technologies. Launched as a collaborative effort among countries, this initiative aims to promote the development and deployment of CCS and CCU (Carbon Capture and Utilization) technologies across the globe.
It facilitates dialogue among member countries, helping to identify and overcome technical, regulatory, and financial barriers to CCS deployment. By sharing best practices and lessons learned, the CCUS Initiative accelerates the global adoption of CCS, making it an essential tool in the climate action arsenal.
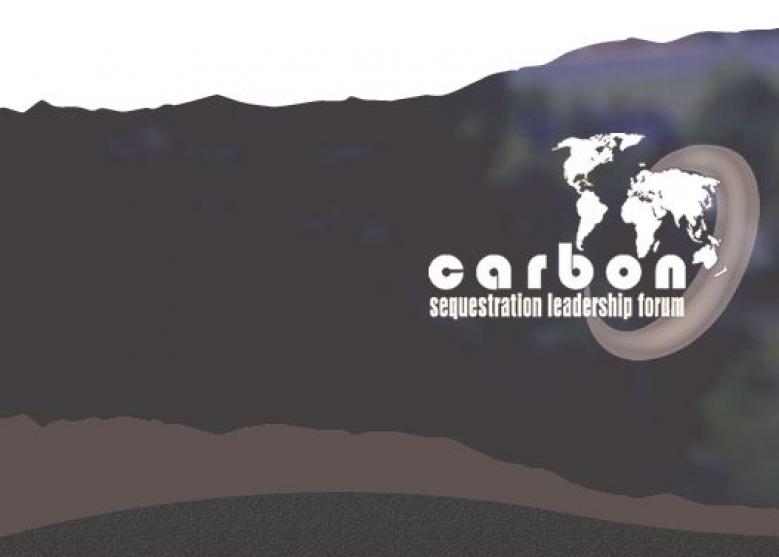
Carbon Sequestration Leadership Forum (CSLF)
The CSLF is a ministerial forum that unites international efforts to develop cost-effective CCS technologies. By bringing together critical industrialized and developing nations, the CSLF focuses on fostering international cooperation in CCS research and development. It addresses the technology’s entire lifecycle, from capture and transport to storage, aiming to make CCS a viable option for reducing global carbon emissions. The forum’s emphasis on policy and regulatory frameworks aids in creating an enabling environment for CCS projects worldwide.
Green Climate Fund (GCF)
As part of the financial mechanisms supporting climate action in developing countries, the GCF recognizes the importance of CCS in mitigating global greenhouse gas emissions. It provides crucial funding for CCS projects, helping to bridge the financial gap that often hinders the deployment of such technologies in lower-income countries. This support not only accelerates the implementation of CCS projects but also ensures that developing nations can contribute to and benefit from global efforts to combat climate change.
Intergovernmental Panel on Climate Change (IPCC)
The IPCC’s assessment reports serve as a scientific foundation for international climate policy, including the role of CCS in mitigating climate change. By highlighting the necessity of CCS in achieving the goals of the Paris Agreement, the IPCC reinforces the urgency of global efforts to research, develop, and deploy CCS technologies. The panel’s work emphasizes that without CCS, the cost and complexity of limiting global warming to agreed targets would increase exponentially.
These international agreements and initiatives collectively forge a path toward a sustainable future by recognizing and supporting the role of CCS in global climate mitigation strategies. Through fostering collaboration, providing financial support, and facilitating knowledge exchange, they highlight the interconnectedness of international efforts to tackle climate change and the indispensable role of innovative technologies like CCS in this endeavour.
THE CARBON PRICING
Carbon pricing is a cornerstone in the global strategy to combat climate change. It is a financial mechanism designed to reduce greenhouse gas emissions by attributing a direct economic cost to emitting carbon dioxide. This system incentivizes businesses and individuals to decrease their carbon footprint through two primary models:
a. carbon taxes and
b.cap-and-trade systems
Carbon Taxes
A carbon tax directly sets a price on carbon by charging emitters a fixed fee per ton of CO2 emitted. This straightforward approach encourages polluters to reduce emissions to save costs. Sweden, for example, introduced a carbon tax in 1991, which has been credited with significantly reducing its greenhouse gas emissions without hindering economic growth. As of 2020, Sweden’s tax stands at approximately $137 per ton of CO2, one of the highest worldwide, and has led to a 26% decrease in emissions from 1990 levels while the economy grew by 78% in the same period.
Cap-and-Trade System
Alternatively, cap-and-trade systems set a maximum (cap) on the total level of greenhouse gas emissions and allow industries with low emissions to sell their extra allowances to more significant emitters (trade). The E.U. Emissions Trading System (EU ETS), the largest and oldest in the world, covers over 11,000 power plants and manufacturing facilities across the E.U. By limiting the number of emissions allowances, the EU ETS has contributed to a significant reduction in emissions across the bloc, achieving a reduction of about 35% in emissions from covered sectors since its inception in 2005.
Tax Incentives for the Carbon Capture and Storage
Tax incentives play a pivotal role in promoting the adoption of CCS technologies by mitigating the high initial costs associated with capture and storage infrastructure. The United States 45Q tax credit is a prime example of such a policy. It offers a tax credit of $50 per metric ton for CO2 stored underground in secure geologic formations and $35 per ton for CO2 utilized in enhanced oil recovery or other end uses. Since its enhancement in 2018, the 45Q tax credit has spurred interest and investment in CCS projects across the country, demonstrating the powerful impact of fiscal incentives on accelerating CCS deployment.
The global landscape of carbon pricing and tax incentives for CCS is diverse and evolving. As of 2021, over 40 countries and 20 cities, states, and provinces have adopted carbon pricing mechanisms, covering about 20% of global greenhouse gas emissions. However, the effectiveness of these policies in promoting CCS and reducing emissions widely varies, depending on the price of carbon and the specific design of the incentives.
Implementing robust carbon pricing mechanisms and offering targeted tax incentives for CCS investments are crucial steps toward realizing the potential of CCS technologies in the global effort to mitigate climate change. By financially motivating the reduction of CO2 emissions and supporting the development of necessary CCS infrastructure, these policies can significantly contribute to achieving international climate targets and transitioning to a low-carbon economy.
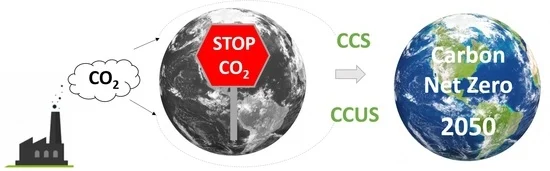
How can Carbon Capture help attain Paris Agreement Goals?
Carbon Capture and Storage (CCS) is heralded as a cornerstone technology in the global strategy to mitigate climate change, playing a crucial role in the ambitious objectives set forth by the Paris Agreement. The agreement, aiming to limit global warming to well below 2 degrees Celsius, with an aspirational target of 1.5 degrees Celsius above pre-industrial levels, necessitates drastic reductions in greenhouse gas emissions across all sectors of the economy. CCS emerges not only as a pivotal solution for reducing current CO2 emissions but also as a vehicle for achieving harmful emissions, essential for balancing the global carbon budget.
The International Energy Agency (IEA) has underscored the necessity of a significant expansion in global CCS capacity to meet the Paris Agreement’s targets. According to IEA projections, achieving net-zero emissions by 2050 requires a nearly 20-fold increase in CCS capacity by 2030. This expansion is critical for decarbonizing sectors where other emission reduction options are either nonexistent or not yet viable at scale, such as in heavy industries (steel, cement, chemicals) and power generation.
Heavy industries are among the most challenging sectors to decarbonize due to their reliance on processes that emit large amounts of CO2. CCS technology captures CO2 emissions at their source, preventing them from entering the atmosphere. For instance, CCS applied to steel production can capture up to 90% of the CO2 emissions generated during the steel-making process. Implementing CCS across these sectors is vital for reducing industrial CO2 emissions, contributing significantly to the overall emission reduction goals of the Paris Agreement.
Beyond merely reducing emissions, CCS is instrumental in generating harmful emissions through processes like Bioenergy with Carbon Capture and Storage (BECCS) and Direct Air Capture (DAC). BECCS involves capturing CO2 from bioenergy production, where biomass is used as fuel and then storing it underground. This process results in net negative emissions, as the biomass growth absorbs CO2 from the atmosphere, which CCS then sequesters. Similarly, DAC technology captures CO2 directly from the atmosphere and stores it, further contributing to negative emissions.
Despite CCS’s clear potential to combat climate change, the deployment of this technology faces several hurdles, including high costs, technological challenges, and the need for supportive policy frameworks. As of now, global CCS capacity is far from the levels required by 2030 to align with the IEA’s net-zero pathway. This gap highlights the urgent need for increased investment, research, and international collaboration to accelerate the development and deployment of CCS technologies.
Therefore, CCS plays an indispensable role in the global effort to meet the Paris Agreement goals. Its capacity to decarbonize hard-to-abate sectors and enable negative emissions positions it as a critical component of the broader climate action strategy. However, realizing the full potential of CCS requires concerted efforts from governments, industries, and the international community to overcome existing barriers and scale up this technology at the pace demanded by the climate crisis.
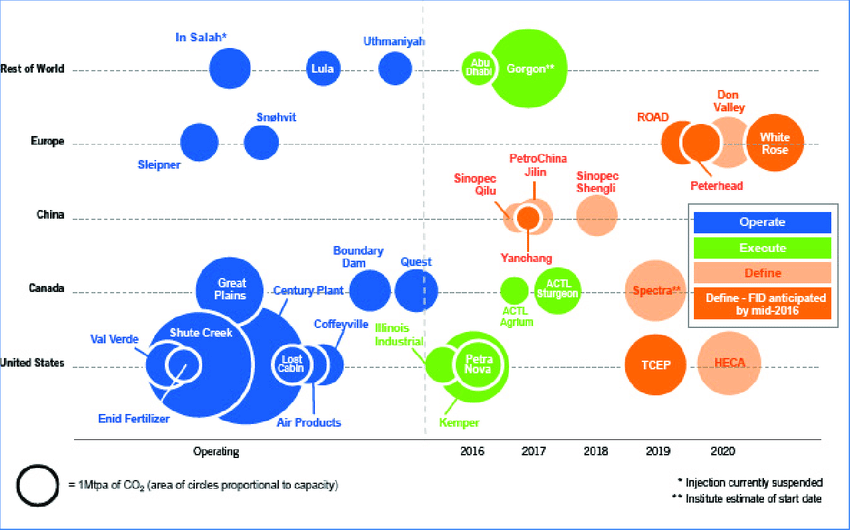
CARBON CAPTURE AND STORAGE POLICY CASES
Exploring the vanguard of Carbon Capture and Storage (CCS) adoption reveals a tapestry of national policies and investments that underscore the technology’s critical role in the global climate agenda.
Let us deep dive into detailed case studies from leading nations, highlighting their strategic approaches and the tangible outcomes of their commitments to CCS development:
United States
The United States has established itself as a leader in CCS technology, significantly propelled by the 45Q tax credit, which offers up to $50 per metric ton for CO2 stored securely underground and $35 per metric ton for CO2 used in enhanced oil recovery or other qualified uses. This incentive has catalyzed numerous projects across the country. Furthermore, the U.S. Department of Energy (DOE) has allocated substantial funds towards CCS research and project development. Notably, projects like the Petra Nova in Texas, one of the world’s largest post-combustion carbon capture projects, have benefited from this supportive policy landscape. The DOE’s continued investment, including a recent $3.5 billion funding announcement for four direct air capture (DAC) hubs, underscores the nation’s dedication to leading in CCS advancements.
Norway
Norway’s pioneering role in CCS began with the Sleipner project in the North Sea, the world’s first offshore CCS operation, capturing over 1 million tons of CO2 annually since 1996. This leadership extends to the Technology Centre Mongstad, a global leader in CCS research and technology testing, and the ambitious Northern Lights project, aiming to store CO2 from industrial sources across Europe. Norway’s commitment is further exemplified by its recent allocation of NOK 16.8 billion (approximately $2 billion USD) to develop full-scale CCS projects, signalling a robust national strategy for carbon capture as a cornerstone of climate action.
Canada
Canada’s approach to CCS is marked by a blend of regulatory measures and strategic investments, particularly in Alberta, the heart of the country’s energy sector. The Carbon Competitiveness Incentive Regulation (CCIR) targets large emitters, encouraging them to reduce carbon intensity through innovations like CCS. Federally, Canada’s investment in CCS projects, including the Boundary Dam project in Saskatchewan, the world’s first post-combustion coal-fired CCS facility, highlights the country’s proactive stance. These efforts are complemented by the recent announcement of a refundable tax credit for carbon capture, utilization, and storage projects starting in 2022, designed to spur further CCS deployment across various sectors.
United Kingdom
The U.K.’s strategic focus on establishing CCS “hubs and clusters” aims to decarbonize industrial regions through coordinated CCS infrastructure and projects. The Carbon Capture and Storage Infrastructure Fund, with an initial allocation of £1 billion, is a testament to this commitment. Projects like the Acorn CCS in Scotland, leveraging existing gas pipelines for CO2 transport and storage, exemplify the U.K.’s integrated approach. This, coupled with the U.K.’s ambition to capture 10 Mt of CO2 annually by 2030, positions the nation as a significant player in CCS deployment.
Australia
The Gorgon CCS project, one of the world’s largest, aims to sequester up to 4 million tons of CO2 annually from natural gas processing. Supported by both government backing and industry collaboration, it showcases Australia’s potential to leverage its vast geological storage capacities for CCS.
Understanding these case studies reflects the growing global consensus on the vital role of CCS in meeting climate goals. While the paths and policies vary, the underlying commitment to investing in CCS technology unites these leading nations. As the world continues to grapple with the complexities of climate change, the strategic deployment of CCS, supported by robust policies and international cooperation, will be paramount in achieving a sustainable and low-carbon future. These examples not only highlight the progress made but also underscore the imperative for continued innovation, investment, and collaboration in the journey toward global climate resilience.
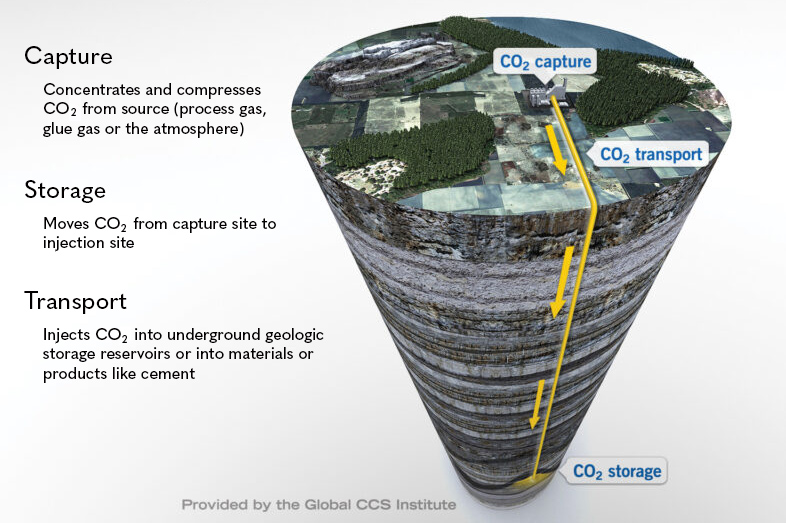
Wrapping up the whole discussion and navigating the complexities of climate change mitigation, the role of Carbon Capture and Storage (CCS) technologies emerges not just as a solution but as a necessity. The detailed exploration of international agreements, carbon pricing, tax incentives, the indispensable role of CCS in meeting Paris Agreement goals, and the policy case studies from leading nations underscores a collective momentum toward a low-carbon future. This journey paved with innovation, strategic investments, and global cooperation, highlights the critical juncture at which we stand today in the fight against climate change.
The ambitious targets set by the Paris Agreement, aiming to limit global warming to well below 2 degrees Celsius, underscore the urgent need for widespread adoption and scaling of CCS technologies. With the International Energy Agency (IEA) indicating that global CCS capacity must increase nearly 20-fold by 2030, the imperative for action is clear. Nations like the United States, Norway, Canada, and the United Kingdom, each with their unique policy frameworks and investments, illustrate the varied paths to harnessing CCS’s potential. Yet, they share a common vision of a carbon-neutral future.
From the pioneering Sleipner project in Norway to innovative tax incentives like the 45Q credit in the United States, these examples demonstrate not only CCS’s feasibility but also its vital role in the broader climate action framework. However, the journey is far from complete. While the current global CCS capacity is growing, it remains insufficient to meet the ambitious climate targets ahead.
The narrative woven by these insights offers both a roadmap and a call to action in future. The convergence of policy support, technological advancement, and international collaboration presents a powerful catalyst for CCS deployment. Yet, the acceleration of these efforts is paramount. The challenge before us is not just technological or financial but fundamentally one of collective will and vision.
In contemplating the path forward, economies are at a pivotal moment in history. The decisions made today, the investments allocated, and the policies enacted will echo through generations. Furthermore, when the forces of innovation, policy, and global cooperation toward CCS and other sustainable technologies merge, the knowledge that our actions today carve out the future of our planet.
In this critical endeavour, the examples set by leading nations, the frameworks established by international agreements, and the mechanisms of carbon pricing and incentives serve as beacons of progress. They remind us that while the challenge of climate change is immense, so too is our capacity for ingenuity, collaboration, and action. The journey toward a sustainable future is complex, demanding, and uncharted, but it is also filled with potential and promise. As we forge ahead, let the lessons learned and the successes achieved illuminate the path toward a world where technology, policy, and collective action converge to create a sustainable, resilient, and carbon-neutral future for all.